University research lab uses Simcenter FLOEFD to design tailless drone
CFD simulation and wind tunnel test correlation for a tailless multi-variant sUAS
NIAR - Wichita State University
The National Institute for Aviation Research (NIAR) at Wichita State University has made a name for itself as the most capable university-based aviation research center in the United States. NIAR provides research, design, testing, certification and training to the aviation manufacturing industry, government agencies, educational entities and other clients that can benefit from their services.
https://www.wichita.edu/research/NIAR/- Centrala:
- Wichita, Kansas, United States
- Produkty:
- Simcenter Products, Simcenter FLOEFD
Simcenter FLOEFD is the go-to tool for NIAR and has been used on numerous projects.
National Institute for Aviation Research - Wichita State University
Research takes flight with Simcenter
Small unmanned aerial systems (sUAS) are a unique class of drones that have a maximum weight, including payload, of 55 pounds (lbs.) and are restricted to fly under 100 miles per hour (mph). This type of drone is becoming very popular for commercial uses such as aerial photography in real estate, and inspection of power poles and cellular towers. In addition, large-scale package delivery companies such as Amazon and the United Postal Service (UPS) are investigating drone use.
The National Institute for Aviation Research (NIAR) at Wichita State University in Wichita, Kansas, recently designed a sUAS to act as a technology demonstrator to highlight NIAR’s capabilities in sUAS design analysis and manufacturing. The design specification NIAR chose is for the sUAS to cruise at 50 mph with a maximum take-off weight of 55 lbs. The drone uses electric propulsion for vertical take-off (VTOL) flight and an internal combustion engine with pusher propeller for forward flight. Multiple design iterations were carried out on a parametric computer-aided design (CAD) model to aerodynamically design the wings.
A one-third scale wind tunnel model was fabricated using additive manufacturing techniques and was tested at the NIAR Walter H. Beech wind tunnel. Computational fluid dynamics (CFD) results and wind tunnel test results were compared. Additionally, a coupled CFD-thermal analysis was conducted to understand the air-cooled internal combustion engine’s cooling performance.
The results showed improvement in the cooling performance of the sUAS’ aircooled internal combustion engine. The aerodynamic design of sUAS was inspired by the following stakeholder requirements:
Tailless, multi-variant configuration
The ability to perform search and rescue missions, and drop a payload of five lbs. and reach a cruise velocity of 50 mph
Forward flight endurance of five hours
Maximum takeoff weight of 55 lbs. to qualify for the Federal Aviation Administration (FAA) Part 107 certification category
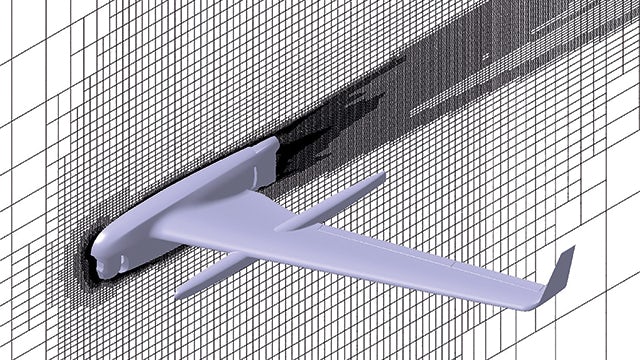
Figure 1: Cartesian Mesh used in the numerical model for the sUAS.
CAD-embedded functionality speeds up production
NIAR used Siemens Digital Industries Software’s Simcenter™ FLOEFD™ software to aerodynamically design the sUAS. NIAR felt Simcenter FLOEFD offered many advantages over other CFD codes. Its CAD-embedded functionality allows for automatic detection of fluid regions and eliminates the need to modify or clean up the geometry. Therefore, the lead time for evaluating multiple design iterations is shorter. And the immersed-body Cartesian mesh allows for quick mesh building for any complex geometry.
The parametric study of the design for different combinations of airfoils, sweep and incidence angles and dihedral angles was carried out to optimize aerodynamic performance and achieve a stable configuration.
Simcenter FLOEFD is based on the FavreAveraged Navier-Stokes model. The governing equations are discretized using the finite-volume method. NIAR used the pressure-based solver in Simcenter FLOEFD in this study. It is based on an implicit scheme with second order accuracy of spatial derivatives and first order accuracy of time derivatives. The flow was computed to be fully turbulent and the modified k-e two-equation turbulence model was used. The Cartesian mesh used in this study is shown in figure 1. The pressure distribution and Mach Number contour are shown in figure 2.
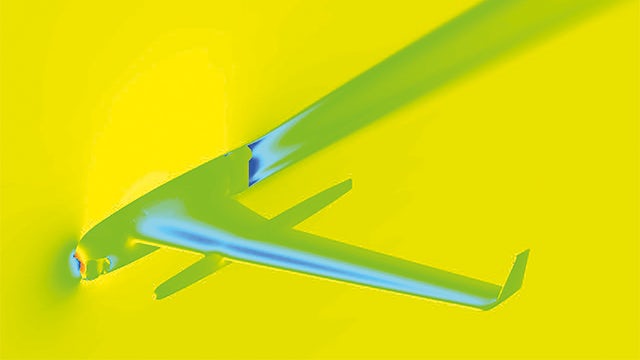
Figure 2: Mach No. contour and surface pressure distribution at velocity = 50 mph, angle of attack = 0°
The wind tunnel model was 3D printed out of PC-ISO in the Fortus 400 printer. It is printed at one-third scale to fit within the printer’s limits. The wind tunnel test was performed at the NIAR Walter H. Beech wind tunnel. It is a subsonic, closed return and atmospheric type with a test section of 7 feet by 10 feet in cross sectional dimensions. The model configuration is a sting mount with internal balance. The model with the sting mount is shown in figure 3.
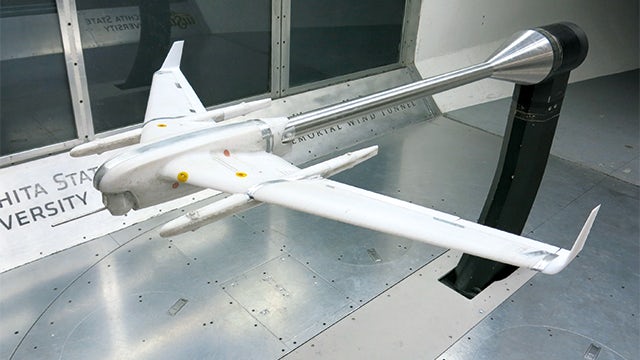
Figure 3: Mach Number contour and surface pressure distribution at velocity = 50 mph, angle of attack = 0°
CFD simulations were performed at cruise conditions (velocity 50 mph, Reynolds number 750,000). Boundary layers were resolved using the modified wall functions approach in Simcenter FLOEFD. Three methods were available: 1) thin boundary layer approach, which is based on the integral boundary layer method; 2) thick boundary layer, which is based on Van Driest’s velocity profile; and 3) hybrid, which combines the thin and thick boundary layer method. For this study, the thin and hybrid methods were investigated. The comparison of the numerical results with the experimental results is shown in figures 4 and 5.
Examining figure 4, it can be seen that good agreement exists between the Simcenter FLOEFD simulation results and the wind tunnel results for angles of attack between minus 10 to 5 degrees using the thin boundary layer approach. This approach also works well for the sideslip angle sweep as can be seen in figure 5.
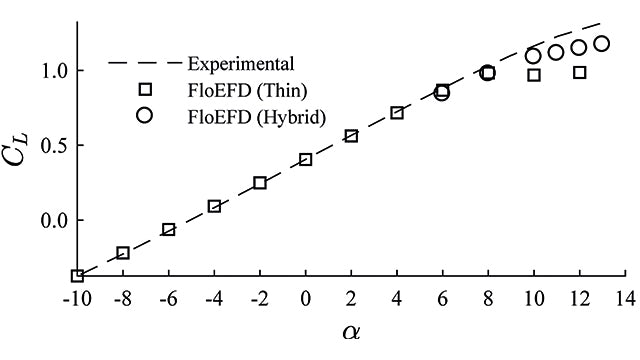
Figure 4: Comparison of lift, drag and pitching moment coefficients.
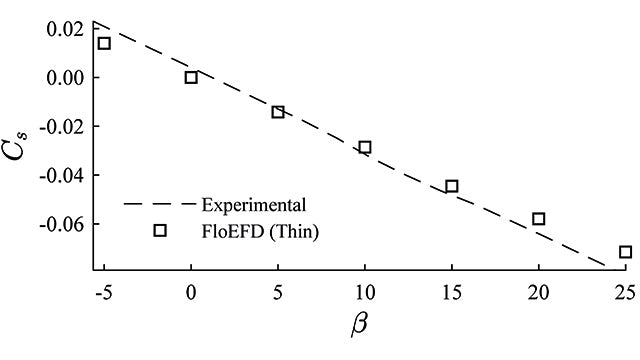
Figure 5: Comparison of side force, yawing and rolling moment coefficients
The hybrid boundary layer approach offers improvement in lift prediction at high angles of attack. Drag prediction at higher angles need further investigation as there was a significant deviation from test.
The thermal management of the sUAS internal combustion engine was the second focus of the CFD analysis. A conjugate heat transfer (CHT) analysis was performed to design ducts for improving cooling efficiency of the sUAS internal combustion engine. The CHT analysis is based on heat transfer (Fourier’s Law, Newton’s Law of Cooling) due to conduction in solids and heat transfer due to convection in fluids (Navier-Stokes equations).
“The ability to do this in Simcenter FLOEFD provides a powerful tool to the design engineer where this would normally have to be left to the CFD analyst,” says Harsh Shah, research engineer, NIAR, Wichita State University.
The comparison of the temperature distribution of the engine assembly with and without the ducts is shown in figure 6. A reduction of approximately 17 percent in maximum temperature of the engine assembly is obtained with ducts and vents incorporated in the assembly.
“Simcenter FLOEFD was a valuable tool when designing the demonstrator sUAS for NIAR,” says Shah. “It allowed multiple design iterations to be performed on both the aerodynamic design of the drone as well as optimizing the cooling strategy for the internal combustion engine. Simcenter FLOEFD is the go-to tool for NIAR and has been used on numerous projects.”
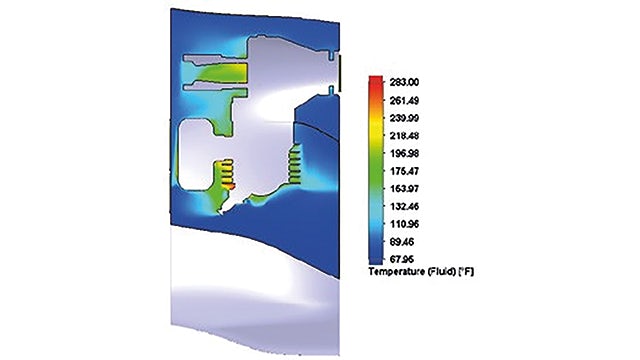
Figure 6: Comparison of temperature distribution (fluid and solid) for the engine assembly with and without ducts.
The ability to do this in Simcenter FLOEFD provides a powerful tool to the design engineer where this would normally have to be left to the CFD analyst.
National Institute for Aviation Research - Wichita State University