Startup uses Simcenter Engineering Services and Simcenter STAR-CCM+ to develop a heat pump that reduces energy costs
Siemens Digital Industries Software services and solution enable ThermoLift to integrate automated design optimization, allowing simulation to drive the design process
ThermoLift
ThermoLift is based out of the Advanced Energy Center at the State University of New York (SUNY) at Stony Brook, has 20 full-time employees and has raised nearly $20 million in funding. The ThermoLift system is a natural gas-driven air conditioner and heat pump that can replace building heating, cooling and hot-water systems with a single appliance.
http://www.tm-lift.com/- Headquarters:
- Stony Brook, New York, United States
- Products:
- Simcenter Products, Simcenter STAR-CCM+
- Industry Sector:
- Industrial machinery
A “crying shame” no more
Most American homes are heated with either a furnace or a boiler, and a small fraction of homes employ heat pumps that use energy (typically electricity) to move ambient heat from outside to inside. High efficiency furnace and boiler systems remain expensive and require low heating temperatures to maintain maximum efficiency; under high heat load conditions, the efficiency will often drop. Electric heat pumps have been available for many years, however, they have not received widespread acceptance due to poor performance in cold climates. Efficiency decreases at low temperatures when heat is needed most, which requires supplemental fuel or resistance heating that can increase costs.
Air conditioners (A/C) are heat pumps that transfer heat from colder to hotter environments. The vast majority of A/C systems use a vapor-compression cycle with a small installation of absorption systems, and represent the largest portion of electricity consumed in a home (22 percent). A/C systems are primarily electrically driven and run during the summer months, thus requiring electricity when it is least available and most expensive. Vapor-compression systems also use refrigerants that present a variety of environmental concerns, including ozone depletion and greenhouse effects.
ThermoLift started as a conversation between friends and colleagues in other ventures, Dr. Peter Hofbauer and Paul Schwartz, in early 2012. The two were discussing a heating, ventilation, air conditioning (HVAC) technology that could change the way buildings use energy. Hofbauer lamented, “It is a crying shame the technology isn’t being developed.” That was the catalyst for Schwartz to start working in his Long Island, New York basement to begin the business development stage for identifying seed capital to create ThermoLift.
Today ThermoLift is based out of the Advanced Energy Center at the State University of New York (SUNY) at Stony Brook, has 20 full-time employees and has raised nearly $20 million in funding, including grants from both the U.S. Department of Energy (DOE) and the New York State Energy Research and Development Authority (NYSERDA). The ThermoLift system is a natural gas-driven air conditioner and heat pump that can replace building heating, cooling and hotwater systems with a single appliance. This appliance is related to a Vuilleumier Heat Pump (VHP), yet uses a modified cycle, the TC-Cycle™, which further enhances the benefits over a VHP, which reduces building heating and cooling energy consumption costs and greenhouse gas emissions.
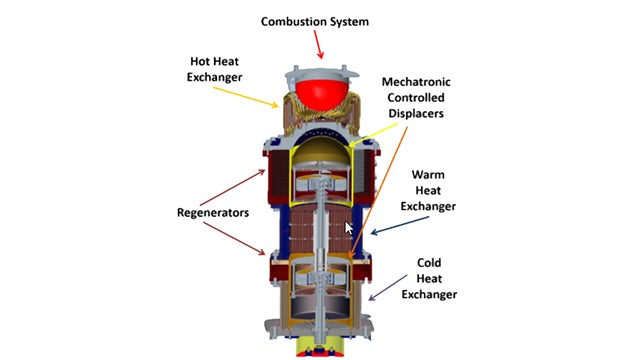
Figure 2: ThermoLift’s innovative generation 1.0 heat pump.
Vuilleumier heat pumps
ThermoLift technology grew out of the VHP, which is a heat engine directly coupled to a heat pump. A VHP moves a working gas, such as helium, between three chambers within a closed system. In the VHP, two displacers that are crank-synchronized reciprocate within a cylinder to move the working gas between separate hot, warm and cold chambers. The thermodynamic cycle uses cooling by expanding gases to create a cold temperature in the cold chamber. Energy from thermal compression and combustion of a fuel (or solar collection) in the hot end to provides warm temperatures in the warm chamber, which can be used for space heating and/ or hot water supply. The ThermoLift device is different from the VHP because the displacers are independently controlled via mechatronics to better control displacer movement and, hence, the thermodynamic cycle.
This temperature difference between the cold chamber and ambient, even at low ambient temperatures, can be exploited to extract heat from the environment for space heating and hot water heating. ThermoLift’s heat pump is an extremely efficient way of capturing heat energy, as opposed to traditional heat pumps, which use electricity, high quality energy that has been generated from thermal energy to develop heat, a low-quality energy. ThermoLift’s heat pump uses heat only and avoids the energy losses associated with the conversion, distribution and phase changes of conventional compressor-based heat pump systems
ThermoLift’s heat pump uses the TC-Cycle to incorporate several innovations to improve performance and reduce costs. The first-generation prototype of this system has already been designed, built and tested. It includes innovations such as an ultra-low-emission combustion burner, electronically controlled actuators for cycle efficiency improvement and innovative heat exchangers. With these improvements, the next-generation VHP device is expected to outperform currently available state-of-the-art HVAC and domestic hot water (DHW) equipment, and will significantly increase operational efficiencies of the thermodynamic process while reducing costs and carbon-dioxide emissions
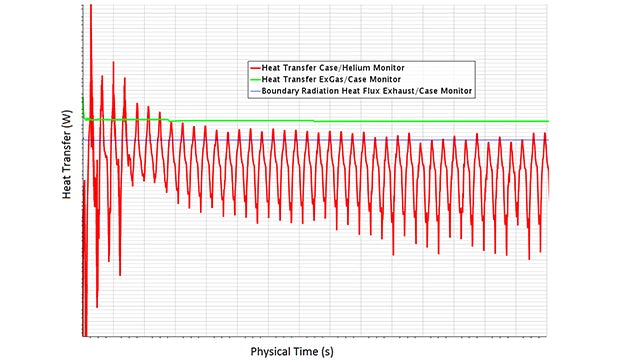
Figure 4: The time history plot of critical heat transfer monitors.
First-generation build testing and data
ThermoLift has built and tested three generations of its TC-Cycle prototype as part of previous DOE and NYSERDA grant programs. ThermoLift’s generation (Gen) 1.0 development focused on the design of an electromechanical drive as an alternative to the crank-synchronized VHPs, which had cost and durability concerns and compromised system thermodynamics due to limited control over displacer movement. Using the acquired knowledge from Gen 1.0, ThermoLift completed development of the Gen 2.0 prototype, which was demonstrated to Oak Ridge National Laboratory (ORNL)/National Renewable energy Laboratory (NREL) representatives in October 2016. ThermoLift’s Gen 3.0 prototype was successfully tested by ORNL in August 2018.
The increased quality and reliability of the data from the Gen 2.0 unit has facilitated simulations and performance. In July 2017, ThermoLift achieved a significant technical milestone, referred to as soft landing of the displacers, as they reciprocated from end to end, which has eliminated machine noise and enabled reliable and continuous operational runtimes (more than 10 hours). ThermoLift expects to assemble multiple Gen 3.0 prototypes and demonstrate high-fidelity performance in laboratory and alpha demonstrations in 2018.
Expanding into the simulation-driven design process
In 2016, ThermoLift started working with Siemens Digital Industries Software to expand into the simulation-driven design process. When developing a new innovative technology like ThermoLift, early prototype development can become expensive when relying on experimental test data. In the early development stage, many conceptual designs have to be evaluated, often with different fundamental ideas being tested. Building and testing these prototypes can present significant risks for a startup. It requires heavy investment in resources and facilities with an uncertain return.
Simulation can provide quick and cost-effective answers. Multiple design concepts and their key performance indexes can be evaluated and poorly performing designs can be eliminated prior to manufacturing a prototype.
Designing and simulating complex systems can be expensive and tricky. Engineers around the world follow the V-Cycle (figure 2) for digital product development. The idea is to have different levels of detail and complexity in the design process, from overall concept and modules to single components. At the beginning stands a broad conceptual design of the system. The entire system consists of several modules like heat exchangers, regenerators and displacers. The most detailed level is the component level, which involves the design of small components like nozzles, orifices or heat exchanger fins.
During this process, designs are created from the top down; from the basic layout to the design of modules and components. The verification process using simulation works in the opposite direction when components and modules are verified and optimized, which results in an improved overall system. In other words, if the modules that make up a system work better, the overall system will work better.
ThermoLift and Simcenter™ Engineering Services adopted this approach to significantly reduce development time and save on prototyping. Up until 2016, the development team at ThermoLift had carried out extensive 1D simulations of their unique thermodynamic cycle. The question was how to predict the physical processes in full 3D. One of the critical modules of the ThermoLift heat pump is the hot heat exchanger. This is the only subsystem in which energy from fossil fuels is entering the system, and it is critical to achieve high efficiency.
The more heat that can be transferred to the helium gas inside, the higher the overall efficiency (figure 1). It is critical to gain full insight into the physics and the path of energy from the burner to the hot helium side, capturing combustion, radiation, convective and conjugate heat transfer. Furthermore, the reciprocating hot displacer introduces a moving domain, which demands a transient analysis requiring larger high-performance computing resources. Finding a simulation solution to model the complex physics combined with moving domains was the challenge, and Simcenter Engineering Services, using Simcenter STAR-CCM+™ software, a Simcenter portfolio product, provided the answer. Simcenter STAR-CCM+ is an all-in-one solution that delivers accurate and efficient multidisciplinary simulation in a single integrated user interface.
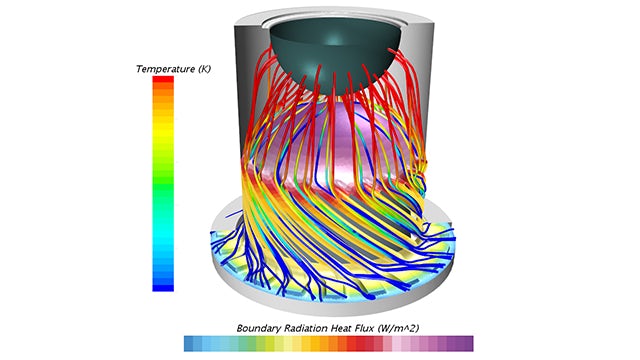
Figure 5: Radiation heat flux distribution and streamlines.
Transient hot heat exchanger analysis
The main objective of this analysis was to give detailed insight into the heat transfer from the burner to the hot helium side. This required modeling radiation, convection and conduction in the burner chamber and hot heat exchanger within the hot helium chamber and the reciprocating displacer motion. A conjugate heat transfer computational fluid dynamics (CFD) model was built from the native Pro/E® software computer-aided design (CAD) data. The unique overset mesh technology of Simcenter STAR-CCM+ was used to model the reciprocating displacer motion. All modes of heat transfer were modeled and all fluids were treated as ideal gas. Cyclic boundary conditions from 1D simulations were applied at the helium outlet, where the hot heat exchanger connects to the first regenerator stage.
There is a combination of transient and steady processes in the system. Although the combustion burner delivers constant heat, the heat transfer into the helium is a cyclic process driven by the reciprocating displacer. As the system heats up, a quasisteady state is eventually achieved in which the heat transfer is balanced over each repeating cycle. This requires long transient simulations, which were completed with Siemens Digital Industries Software’s highperformance computer clusters as part of this cooperation project. Figure 4 shows the time history of the heat transfer across critical component interfaces from the burner to Helium. After 15-to-20 cycles, the heat transfers become cyclic and cycleaveraged performance data could be derived. The data could predict key performance indicators such as heat exchanger efficiency and heat loss to evaluate this Gen 2 design.
Figure 5 shows the volume-rendered temperature distribution of the exhaust gas and helium inside the machine when the displacer is moving downwards. Figure 6 shows the distribution of the boundary radiation heat flux on the hot heat exchanger facing the burner. The streamlines show the path of the exhaust gas from the burner through the spiral channel design colored by temperature, providing insight into where the gas is losing temperature to the hot heat exchanger.
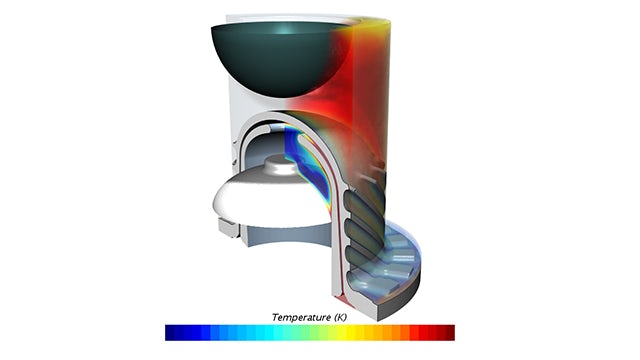
Figure 6: Volume-rendered temperature distribution of exhaust gas and helium.
Partnering with Simcenter Engineering Services
The results from the transient hot heat exchanger analysis carried out by Simcenter Engineering Services helped the design team at ThermoLift to identify critical performance data, including problems and causes:
Exhaust gas temperature was too high when leaving the heat exchanger. This led to a pre-combustion heater that recovered this excess heat back into the system
Total heat transfer into the helium was too low to meet performance targets. A more complex tubular heat exchanger has been developed (figure 1)
The radiation heat transfer into the heat exchanger was a critical contributor to overall heat input
Potentially changing the way buildings use energy
Siemens Digital Industries Software and ThermoLift are continuing their partnership to further expand the firm’s simulation-driven design process. With continued technology transfer and the support of Simcenter Engineering Services, the ThermoLift simulation team has become experienced Simcenter STAR-CCM+ users, simulating and developing components every day. Siemens supports and develops a roadmap to target continuous growth and process improvement at ThermoLift by:
Integrating more components into the simulation. The aim is to model the entire machine in 3D
Integrating automated design optimization. Instead of just evaluating chosen designs, they can let simulation drive the design process
Providing analysis services and high-performance computing resources for big simulations, keeping the costs down while still thinking big
ThermoLift’s technology can potentially reduce building heating and cooling energy consumption and costs, and associated greenhouse gas emissions. That could change the way buildings use energy in the future and make it a greener world.